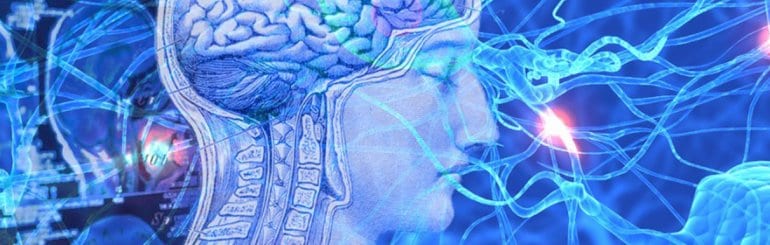
The Future of Protein Biomarker Detection Methods in Neuropathology
Progress in biomarker detection methods have enabled exploration, identification, and monitoring of protein markers in a wide range of tissues and biofluids. Nevertheless, as the blood-brain barrier restricts access to the brain and considering that neurological biomarkers are present at extremely low concentrations in blood, access to advanced, ultra-sensitive biomarker identification techniques is imperative for advancing neurology research.1
What are the current state-of-the-art methods? What does the future of neuropathology hold?
Scroll to:
● Conventional and emerging technologies for protein biomarker detection
● Imaging biomarkers — They need a partner to enhance diagnostics
● Fluid biomarker detection — The path to accessible diagnostics
● Biofluid-based biomarker detection methods
● Simoa® technology — Ultrasensitive digital ELISA
Protein biomarker detection in neuropathology
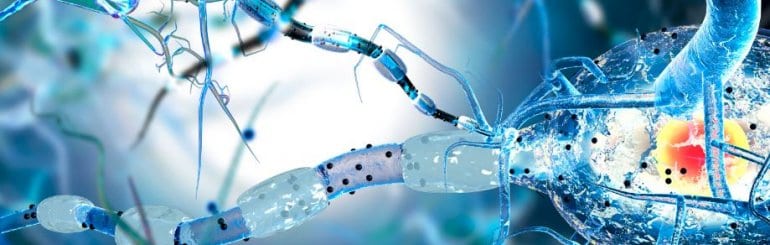
Diagnosis of neuropathological diseases relies on accurate biomarker detection methods as well as the continued expansion and development of improved ultrasensitive identification techniques. Major advancements in omics technologies, specifically in proteomics with mass spectrometry as an analytical tool, have fueled biofluid and tissue biomarker identification for a wide range of pathologies.2,3,4,5 Recent technology developments permitting non-invasive protein biomarker detection offer researchers new opportunities to investigate novel or difficult-to-detect proteins, opening up promising new perspectives for earlier disease detection.
Conventional and emerging technologies for protein biomarker detection
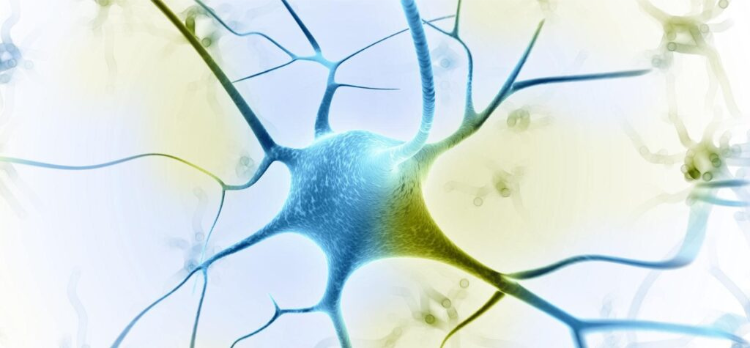
Imaging biomarkers — Better together to improve diagnostics
There is an increasing need for a multidisciplinary approach to improve the accuracy and efficiency of neuropathology diagnosis. The field of neuropathology benefits from the collaborative use of well-established imaging techniques together with novel imaging biomarkers.6 However, these technologies are expensive, which increases the costs of clinical trials. Availability is also limited to specialized centers, which hampers widespread accessibility with patients.
Positron emission tomography (PET) and Alzheimer’s Disease (AD)
PET imaging can assess abnormal levels of amyloid-beta (Aβ) protein in the brain, constituting an important step in the identification of individuals at high risk of developing Alzheimer’s disease, as well as potential future candidates for anti-Aβ therapies.7
PET imaging also allows the detection of tau, a neuronal microtubule-associated protein, in the brain.8 Although a comprehensive validation of the second-generation tau tracers remains to be completed before incorporation into the clinic, tau PET is emerging as a powerful imaging tool for diagnosing cognitive impairments in patients.8-10 Recently, other PET ligands that target the synaptic vesicle glycoprotein 2A (SV2A)11,12 that allow for the measurement of synaptic density were included in preclinical studies and ongoing clinical trials targeting different neurological conditions, including AD.
PET is a gold standard but remains expensive and inaccessible for AD diagnosis
To increase the accessibility of AD diagnosis and offer a path to less expensive clinical trials, patients, researchers and clinicians need screening tools that help inform when PET imaging is needed. Fluid biomarkers are a promising route to accessible and cost-effective AD detection.13
Magnetic resonance imaging (MRI) and multiple sclerosis (MS)
As a non-invasive imaging tool, MRI is the preferred method for diagnosing MS. Through a system of magnetic fields and radio waves, MRI measures the relative water content in different tissues in the body.As a result of the immune system attacking the fatty myelin layer that protects nerve cells, the MRI scan highlights demyelinated lesions by displaying a higher level of water retention.14
The development of emerging MRI techniques allows for greater imaging resolution and provides valuable information to aid in the diagnosis and categorization of neurological conditions. 7T structural MRI can detect biochemical changes in the brain of MS patients very early in the disease15. Additionally, this technique provides high spatial resolution volumetry measurements of hippocampal subfields, crucial in neurodegenerative disorders and epilepsy.16
Researchers and health practitioners can also use functional MRI (fMRI) to measure neuronal dysfunction indirectly. Several neuropathologies, including autism, strokes, and neurodegenerative diseases, are being studied with fMRI techniques to detect neural circuit defects. Although further extensive research is required prior to their implementation in clinical diagnosis, these new techniques can improve our understanding of how neural networks function and provide better insight regarding neuropathologies in the future.
MRI remains expensive and is not easily accessible for diagnosis and staging of MS Although MRI has a major role in establishing the diagnosis of MS, there is an urgent need for cost-effective pre-screening methods to identify and prioritize patients who would benefit from a detailed view of the lesions. Neuro-biomarker detection in biofluids opens the doors to cheaper, more accessible alternatives for MS diagnosis and staging.
Computed tomography (CT) and traumatic brain injury (TBI)
Quicker than MRI, CT imaging is the preferred diagnostic tool for brain injury, using intracranial hemorrhage as a biomarker for acute TBI detection. Based on differences in contrast between tissues and structures, CT allows the visualization of skull fractures, patterns of atrophy and evidence of internal bleeding.
CT has continually improved over the past decades, with emerging technologies showing encouraging results for better, more precise imaging. Contrary to conventional CT imaging, dark-field CT uses small-angle scattering characteristics and X-ray tissue refraction to produce highly contrasted images of very fine tissue structures.17 While not in clinical use yet, animal studies demonstrate its potential in diagnosing and staging diseases. We still need further research to determine its utility for TBI.
Despite CT’s long history and progress, its levels of radiation and associated carcinogenic potential, especially in regard to children, is a pressing concern.18 Furthermore, repetitive CT exams represent a non-negligible financial burden for patients, limiting their capacity to access this routine procedure. CT remains a valuable diagnostic procedure, but advancements in biomarker detection methods for TBI are needed to ensure patient safety and care accessibility without compromising image quality.
Fluid biomarker detection — The path to accessible diagnostics
Cerebrospinal fluid (CSF)
Associated with several neurological conditions, detectable CSF biomarkers constitute a powerful detection tool and a significant addition to imaging biomarkers in neuropathology.
Hallmarks of AD accessible through CSF, Aβ, total (t-)Tau, and phosphorylated (p-)Tau, were included as biomarkers in the 2018 NIA-AA AT(N) framework along with standard neuroimaging procedures.19 Although AT(N) biomarker profiling is currently the sole accepted classification system for AD biological investigation, ongoing research has identified novel promising CSF biomarkers including new phosphorylated forms of tau, neurofilament light chain (NfL), glial fibrillary acidic protein (GFAP), and synaptosomal-associated proteins (SNAPs), further expanding the list of potential actors in early disease detection.20,21
Lumbar puncture is the preferred method for CSF sample collection. However, this costly, invasive procedure puts patients at risk of complications, making it unsuitable for most primary and secondary care settings. This inaccessibility has left 50-70% of symptomatic AD patients without a correct and timely diagnosis, impacting quality of life, medical autonomy, and general wellbeing.22
While providing valuable neurological data that is vital for early AD detection and prognosis, limitations surrounding CSF collection emphasizes the importance of quickly developing alternative biomarker detection methods.
Blood
Blood-based biomarker detection methods could offer a non-invasive option for diagnostics and disease monitoring. Quick, cost-effective, and reliable, blood-based biomarkers are ideal for pre-screenings before more intensive imaging.
Although blood biomarker identification techniques are still emerging, neuronal proteins such as NfL chains can already be detected in the blood through the use of ultrasensitive instruments23. Measuring NfL levels alongside with MRI can significantly improve the detection of disease activity in MS prior to a relapse.
A larger range of blood-based diagnostic tests is being developed for other neurological conditions to facilitate diagnosis and prognosis while avoiding invasive and risky procedures like CSF collection. Blood biomarkers such as lysosomal enzymes and α-synuclein species have been identified as closely reflecting Parkinson’s pathophysiology, though current diagnostic criteria have yet to incorporate them.24 On the other hand, blood biomarkers in AD, such as pTau181 in plasma samples, are already considered in the design of future clinical trials,25 underscoring the importance of further developing ultrasensitive blood-based biomarker detection methods.
Other biofluids
Saliva is an easily accessible biofluid that has gained increased attention for diagnostics in the last few years, including in a number of neurological disorders.26 Human salivary Raman fingerprint was reported as a novel biomarker for the diagnosis of neurodegenerative Amyotrophic Lateral Sclerosis (ALS).27 Salivary t-tau, GFAP, and lactoferrin are all promising biomarkers for AD diagnostics in saliva.28,29 Urine is another biofluid that has demonstrated promise as a source of diagnostic information for neuropathology.30-31
Learn how Quanterix is advancing biofluid biomarker detection methods for neurology
Biofluid-based biomarker detection methods
Ultrasensitive biofluid biomarker detection methods are primarily immunoassays, building off traditional enzyme-linked immunosorbent assays (ELISA) as well as flow cytometry-based detection methods. Advancements in technologies are enabling new study designs and diagnostic potential through new or improved capabilities:
- Multiplex technologies: profile multiple analytes simultaneously in a single sample
- Femtogram-level sensitivity: detect protein biomarkers at ultralow levels to explore new pathological pathways or non-invasively measure biomarkers of interest
- Automation: increase throughput and minimize manual labor and errors to improve reliability and productivity across studies
Learn more about digital ELISA biomarker detection methods at Quanterix
SimoaⓇ is expanding opportunities in protein biomarker detection
There have been impressive advancements in immunoassays and biofluid-based biomarker detection methods, delivering increasingly accurate and sensitive biomarker detection. Biofluid biomarker detection technologies are fueling preclinical and clinical research and improving diagnostic capabilities for neuropathology patients.
Quanterix Simoa® Technology’s ultrasensitive and flexible immunoassays offer ultrasensitive biomarker detection in blood, CSF, and other biofluids. The single-molecule array is a novel digital ELISA that is broadly applicable to research in oncology, neurology, cardiology, inflammation, and infectious disease. To date, Simoa® assays have had a notable impact on MS, AD, and TBI research. Simoa® technology is transforming neuropathology research from discovery to the clinic.
Click here to learn about the advancements Quanterix technology has made in Alzheimer’s Disease.
References
- Harris SE, Cox SR, Bell S, et al. Neurology-related protein biomarkers are associated with cognitive ability and brain volume in older age. Nat Commun. 2020;11(1):800. Published 2020 Feb 10. doi:10.1038/s41467-019-14161-7
- Chase Huizar C, Raphael I, Forsthuber TG. Genomic, proteomic, and systems biology approaches in biomarker discovery for multiple sclerosis. Cell Immunol. 2020;358:104219. doi:10.1016/j.cellimm.2020.104219
- Khoo A, Liu LY, Nyalwidhe JO, et al. Proteomic discovery of non-invasive biomarkers of localized prostate cancer using mass spectrometry. Nat Rev Urol. 2021;18(12):707-724. doi:10.1038/s41585-021-00500-1
- Nakayasu ES, Gritsenko M, Piehowski PD, et al. Tutorial: best practices and considerations for mass-spectrometry-based protein biomarker discovery and validation. Nat Protoc. 2021;16(8):3737-3760. doi:10.1038/s41596-021-00566-6
- Isin EM, Turesky RJ. Introduction: Mass Spectrometry and Emerging Technologies for Biomarker Discovery in the Assessment of Human Health and Disease. Chem Res Toxicol. 2016;29(12):1901-1902. doi:10.1021/acs.chemrestox.6b00429
- Young PNE, Estarellas M, Coomans E, et al. Imaging biomarkers in neurodegeneration: current and future practices. Alzheimers Res Ther. 2020;12(1):49. Published 2020 Apr 27. doi:10.1186/s13195-020-00612-7
- Vlassenko AG, Benzinger TL, Morris JC. PET amyloid-beta imaging in preclinical Alzheimer’s disease. Biochim Biophys Acta. 2012;1822(3):370-379. doi:10.1016/j.bbadis.2011.11.005
- Congdon EE, Sigurdsson EM. Tau-targeting therapies for Alzheimer disease. Nat Rev Neurol. 2018;14(7):399-415. doi:10.1038/s41582-018-0013-z
- Bischof GN, Dodich A, Boccardi M, et al. Clinical validity of second-generation tau PET tracers as biomarkers for Alzheimer’s disease in the context of a structured 5-phase development framework. Eur J Nucl Med Mol Imaging. 2021;48(7):2110-2120. doi:10.1007/s00259-020-05156-4
- Wong DF, Comley RA, Kuwabara H, et al. Characterization of 3 Novel Tau Radiopharmaceuticals, 11C-RO-963, 11C-RO-643, and 18F-RO-948, in Healthy Controls and in Alzheimer Subjects [published correction appears in J Nucl Med. 2019 Jan;60(1):141]. J Nucl Med. 2018;59(12):1869-1876. doi:10.2967/jnumed.118.209916
- Cai Z, Li S, Matuskey D, Nabulsi N, Huang Y. PET imaging of synaptic density: A new tool for investigation of neuropsychiatric diseases. Neurosci Lett. 2019;691:44-50. doi:10.1016/j.neulet.2018.07.038
- Toyonaga T, Fesharaki-Zadeh A, Strittmatter SM, Carson RE, Cai Z. PET Imaging of Synaptic Density: Challenges and Opportunities of Synaptic Vesicle Glycoprotein 2A PET in Small Animal Imaging. Front Neurosci. 2022;16:787404. Published 2022 Mar 8. doi:10.3389/fnins.2022.787404
- Contador J, Vargas-Martínez AM, Sánchez-Valle R, Trapero-Bertran M, Lladó A. Cost-effectiveness of Alzheimer’s disease CSF biomarkers and amyloid-PET in early-onset cognitive impairment diagnosis. Eur Arch Psychiatry Clin Neurosci. 2023;273(1):243-252. doi:10.1007/s00406-022-01439-z
- Ziemssen T, Akgün K, Brück W. Molecular biomarkers in multiple sclerosis. J Neuroinflammation. 2019;16(1):272. Published 2019 Dec 23. doi:10.1186/s12974-019-1674-2
- McKiernan EF, O’Brien JT. 7T MRI for neurodegenerative dementias in vivo: a systematic review of the literature. J Neurol Neurosurg Psychiatry. 2017;88(7):564-574. doi:10.1136/jnnp-2016-315022
- Park JE, Cheong EN, Jung DE, Shim WH, Lee JS. Utility of 7 Tesla Magnetic Resonance Imaging in Patients With Epilepsy: A Systematic Review and Meta-Analysis. Front Neurol. 2021;12:621936. Published 2021 Mar 19. doi:10.3389/fneur.2021.621936
- Viermetz M, Gustschin N, Schmid C, et al. Dark-field computed tomography reaches the human scale. Proc Natl Acad Sci U S A. 2022;119(8):e2118799119. doi:10.1073/pnas.2118799119
- Fred HL. Drawbacks and limitations of computed tomography: views from a medical educator. Tex Heart Inst J. 2004;31(4):345-348.
- Jack CR Jr, Bennett DA, Blennow K, et al. NIA-AA Research Framework: Toward a biological definition of Alzheimer’s disease. Alzheimers Dement. 2018;14(4):535-562. doi:10.1016/j.jalz.2018.02.018
- Filippi P, Vestenická V, Siarnik P, et al. Neurofilament light chain and MRI volume parameters as markers of neurodegeneration in multiple sclerosis. Neuro Endocrinol Lett. 2020;41(1):17-26.
- Teunissen CE, Chiu MJ, Yang CC, et al. Plasma Amyloid-β (Aβ42) Correlates with Cerebrospinal Fluid Aβ42 in Alzheimer’s Disease. J Alzheimers Dis. 2018;62(4):1857-1863. doi:10.3233/JAD-170784
- Hansson O, Edelmayer RM, Boxer AL, et al. The Alzheimer’s Association appropriate use recommendations for blood biomarkers in Alzheimer’s disease. Alzheimers Dement. 2022;18(12):2669-2686. doi:10.1002/alz.12756
- Alirezaei Z, Pourhanifeh MH, Borran S, Nejati M, Mirzaei H, Hamblin MR. Neurofilament Light Chain as a Biomarker, and Correlation with Magnetic Resonance Imaging in Diagnosis of CNS-Related Disorders. Mol Neurobiol. 2020;57(1):469-491. doi:10.1007/s12035-019-01698-3
- Parnetti L, Gaetani L, Eusebi P, et al. CSF and blood biomarkers for Parkinson’s disease. Lancet Neurol. 2019;18(6):573-586. doi:10.1016/S1474-4422(19)30024-9
- Tissot C, Therriault J, Kunach P, et al. Comparing tau status determined via plasma pTau181, pTau231 and [18F]MK6240 tau-PET. EBioMedicine. 2022;76:103837. doi:10.1016/j.ebiom.2022.103837
- Farah R, Haraty H, Salame Z, Fares Y, Ojcius DM, Said Sadier N. Salivary biomarkers for the diagnosis and monitoring of neurological diseases. Biomed J. 2018;41(2):63-87. doi:10.1016/j.bj.2018.03.004
- Carlomagno C, Banfi PI, Gualerzi A, et al. Human salivary Raman fingerprint as biomarker for the diagnosis of Amyotrophic Lateral Sclerosis. Sci Rep. 2020;10(1):10175. Published 2020 Jun 23. doi:10.1038/s41598-020-67138-8
- Katsipis G, Tzekaki EE, Tsolaki M, Pantazaki AA. Salivary GFAP as a potential biomarker for diagnosis of mild cognitive impairment and Alzheimer’s disease and its correlation with neuroinflammation and apoptosis. J Neuroimmunol. 2021;361:577744. doi:10.1016/j.jneuroim.2021.577744
- Bermejo-Pareja F, Del Ser T, Valentí M, de la Fuente M, Bartolome F, Carro E. Salivary lactoferrin as biomarker for Alzheimer’s disease: Brain-immunity interactions. Alzheimers Dement. 2020;16(8):1196-1204. doi:10.1002/alz.12107
- Kurbatova N, Garg M, Whiley L, et al. Urinary metabolic phenotyping for Alzheimer’s disease. Sci Rep. 2020;10(1):21745. Published 2020 Dec 10. doi:10.1038/s41598-020-78031-9
- Kohlhase K, Frank F, Wilmes C, et al. Brain-specific biomarkers in urine as a non-invasive approach to monitor neuronal and glial damage. Eur J Neurol. 2023;30(3):729-740. doi:10.1111/ene.15641