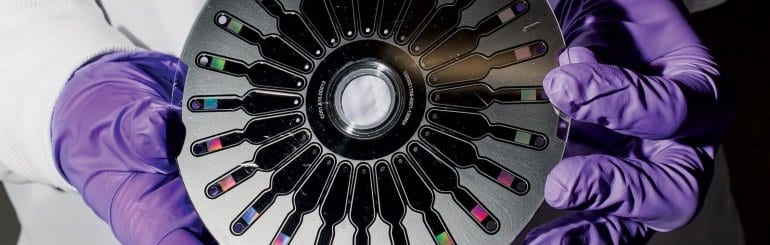
Immunoassay Technologies: Past, Present, and Future
Table of Contents
Is it time to retire your western blot or ELISA equipment? Not necessarily — but you may want to consider supplementing your research with some of the latest immunoassay technologies if you’re looking to detect proteins at ultra-low concentrations, quantify several proteins in a single sample, or ramp up your productivity.
Immunoassay technologies continue to evolve in such a way that a number of assays may be available to meet your research objectives. In order to make the right choice for your lab, it’s important to understand the pros, cons, and capabilities of different types of immunoassays — both traditional and modern.
A quick overview of immunoassay technologies
If you’re well-versed in immunoassays — feel free to skip this section and move on to learning more about the pros, cons, and capabilities of various types of immunoassay technologies.
Immunoassays (IA) are used to measure the presence or concentration of biological molecules in a sample. They’re used in a wide variety of applications including drug discovery, clinical diagnostics, biopharmaceutical analysis, and environmental monitoring.
Immunoassay Principle
Immunoassay technologies take advantage of the body’s natural ability to detect bacteria, viruses, or other foreign substances (known as antigens) and simulate an immune response resulting in the production or secretion of antibodies (Ab) specific to that antigen.
Antibodies are antigen-specific. An antibody’s binding site is so exclusive to the antigen it targets, that it is often compared to a lock and key relationship. By leveraging this relationship, immunoassay technologies use an antibody or an antigen to detect a molecule of interest, known as an analyte.
Classifying Immunoassays
Competitive vs. non-competitive
Immunoassays differ on the type of reaction method used and are either classified as competitive or non-competitive.
Competitive immunoassays limit the number of antigen binding sites, forcing a target analyte and a labeled analog to compete for antibody binding. Since the unlabeled analyte binds more readily than the labeled analog, the amount of bound labeled analyte is inversely proportional to the amount of analyte of interest.
There is also a distinction between competitive immunoassays according to the order in which the analytes are added.
Simultaneous or equilibrium assay – The most common method involves adding all components simultaneously.
Sequential assay – Incubating the sample with the antigen before adding the labeled analog increases sensitivity.
Non-competitive immunoassays have excess antibody binding sites and produce a signal directly proportional to the amount of analyte in the sample.
Use Case: Competitive Immunoassays for the Detection of Small Molecules
Wang et al. developed an ultra-sensitive competitive Simoa immunoassay that was approximately 50-fold more sensitive than conventional ELISA. Read the publication.
Direct vs. Indirect Immunoassays
Immunoassays are considered direct or indirect depending on whether the primary antibody is labeled with a detection medium or whether a secondary labeled antibody is necessary for detection.
Direct Immunoassay – The antigen is detected by a primary antibody, conjugated with a label for detection (enzyme, fluorophore, etc.).
Advantages
- Shorter and simpler protocols
- Only requires one detection antibody
- No problems with secondary antibody cross-reactivity
Disadvantages
- Conjugated primary antibodies may be expensive
- Limited selection of conjugated primary antibodies
- Weaker signal intensity
- Reduced non-specific binding
- Weak antibody specificity may increase background
Indirect Immunoassay – An unconjugated primary antibody binds to the antigen. A secondary antibody conjugated with a detection label binds to the primary antibody.
Advantages
- Inexpensive secondary antibodies
- Amplified signal due to multiple secondaries binding to primary
- Large selection of conjugated secondary antibodies
- Conjugated secondary antibodies can be used to detect multiple primary antibodies
- Labeled secondary antibodies provide options for multiple detection methods
Disadvantages
- Protocols require additional steps which adds complexity
- Nonspecific binding may increase background
Immunoassay Labels for Detection
Immunoassay labels allow for the detection and measurement of antibodies or antigens. Without them, scientists would have no way to detect or quantify their target antigens. Although it’s common to see immunoassay technologies classified by their labels, some types of immunoassays can utilize a variety of labels depending on the assay limitations and the research objectives.
Radioactive – The earliest immunoassays used radioactive isotopes. Because of the health hazard of using radioactive substances, there has been a movement toward safer methods.
Chromogenic – Chromogenic detection is associated with enzyme immunoassays. This enzyme complex produces a precipitate that makes colorimetric changes visible to the naked eye.
Fluorescence – Fluorescently tagged antibodies require the use of an instrument capable of capturing the fluorescent signal. This technique is growing in popularity because it offers the potential to multiplex assays previously limited to detecting one analyte at a time.
Luminescence – Luminescence detection methods use chemiluminescent reporters. An enzyme-antibody reaction produces light as a byproduct. Due to its magnification potential, this is the most sensitive detection method.
The First Immunoassay
Rosalyn Sussman Yalow and Solomon Berson developed radioimmunoassay (RIA) using radioisotopes in the 1950s. They received the Nobel prize, making Yalow the second female Nobel Prize winner. Their assay proved that type 2 diabetes is caused by the body’s inefficient use of insulin and not by a lack of insulin. Despite the precision, RIAs have fallen out of favor because of the difficulties and dangers of working with radioactive substances.
Traditional types of immunoassays remain relevant but have limitations
Western Blot (also called immunoblotting)
Western blotting, developed in 1979 by Towbin, et al. involves the transfer of biological samples from an electrophoresis gel to the surface of a membrane. Following the transfer, the membrane is treated with a blocking buffer to prevent non-specific binding. The analyte is probed with a primary and secondary before visualization. The resulting band size and positioning allow for a qualitative and semi-quantitative analysis of the target analyte as signal intensity correlates with antigen concentration.
The biggest drawback to western blots is the lack of reproducibility. Reactions measured at a steady state are predictable. Western blot results, however, are measured in the middle of a reaction curve and are thus susceptible to researcher-imposed variances. Kinetic differences throughout the assay compound these variances. An extra second of membrane incubation or exposure could dramatically impact the signal intensity. Because of the susceptibility to kinetic influence, western blotting is considered an art and should be used for qualitative and crude quantitative analysis only.
Summary
Sensitivity: Qualitative, semi-quantitative
Reproducibility: Low (High kinetic differences)
Capability: Singleplex
Dynamic Range: Low (Saturation and background are common)
Sample Volume: High (15µl – 700µl depending on well format)
Direct vs. Indirect: Mostly indirect
Competitive vs Non-competitive: Non-competitive
Detection Method: Radioactive, Colorimetric, Fluorescent, Luminescence
Strongest Use Case: Detect the presence of a single protein
Example use cases
- Comparing expression of a target protein from various tissues.
- Crudely visualizing how a protein responds to disease or drug treatment.
- Determining if protein expression is suppressed in a small number of cell lysate samples.
Enzyme Immunoassay and Enzyme-Linked Immunosorbent Assay (ELISA)
Enzyme immunoassays (EIA) use an antibody linked to an enzyme to detect and measure antigens or antibodies.
Enzyme-linked immunosorbent assays (ELISA) are arguably the most common and popular quantitative immunoassay technology. This type of immunoassay employs ELISA microplates to immobilize a target antigen or antibody, bind with a targeted analyte, and measure an emitted signal. There are four main types of ELISAs.
Direct ELISA – A single, enzyme-linked antibody directly binds with an antigen. This is ELISA in its simplest form but suffers from low flexibility and potentially high background signal. Direct ELISAs are useful when analyzing immune responses to antigens.
Indirect ELISA – An enzyme-linked secondary antibody binds with a primary antibody bound to the antigen. The secondary antibodies make this ELISA highly versatile, sensitive, and cost-effective. However, the secondary presents the potential for cross-reactivity and adds complexity to the protocol. Indirect ELISAs are useful when determining total antibody concentration.
Sandwich ELISA – An antigen binds to a primary antibody attached to the plate. A secondary enzyme-linked antibody then attaches to the antigen. This ELISA is highly flexible, sensitive, and specific but requires large antigens of interest to accommodate multiple antibody bindings. Sandwich ELISAs are particularly useful when analyzing complex samples, since the antigen doesn’t need to undergo purification before measurement.
Competitive ELISA – The sample antibodies compete with enzyme-linked antibodies to bind with the antigen. Since the sample antibodies outcompete the enzyme-linked antibodies, the amount of signal is inversely correlated to the target antigen. Although competitive ELISAs are relatively complex and require an inhibitor antigen, they are ideal for low-concentration samples.
Because ELISA’s are widely used, a large selection of validated assays and kits are available on the market. While initially, ELISAs took 2 days to perform, were limited to singleplex detection assays, and required a large amount of sample, new immunoassay technologies have paved the way for faster and cheaper assays that deliver more information.
Summary
Sensitivity: Quantitative
Reproducibility: Medium (Depending on detection method and measurement window)
Capability: Singleplex
Dynamic Range: Medium (Lose linearity over a few orders of magnitude)
Sample Volume: Medium (100µl)
Direct vs. Indirect: Either
Competitive vs Non-competitive: Either
Detection Method: Colorimetric, Fluorescent, Luminescence
Strongest Use Case: Quantify a single protein
Example use cases
- Diagnosing infectious conditions like HIV, Lyme disease, and toxoplasmosis through antibody detection.
- Detecting and quantifying peptides, proteins, antibodies, and hormones for biomedical and pharmacological research.
- Non-invasive prescreening tools for detecting cerebral amyloidosis in plasma in clinical trials Read the publication.
Bead-based Immunoassay
Bead-based immunoassays use antibody-coated beads to detect analytes of interest. Beads are distinguished by color, fluorescence, luminescence, or bead size. Detection with fluorescent-labeled beads allows for robust multiplexing.
This immunoassay technology allows for highly quantitative multiplex detection of multiple analytes within a single sample. In combination with some read-out technologies, the sensitivity attainable in bead-based assays can be several orders of magnitude higher than what’s possible in standard ELISA formats, thus reducing workflow and sample volume while maximizing data output.
Summary
Sensitivity: Quantitative
Reproducibility: High
Capability: Singleplex or Multiplex
Dynamic Range: High (Maintain linearity over three to five orders of magnitude)
Sample Volume: Low (25µl – 150µl, protocols vary)
Direct vs. Indirect: Either
Competitive vs Non-competitive: Competitive
Detection Method: Colorimetric, Fluorescent, Luminescence, Bead Size
Strongest Use Case: Quantify multiple proteins or quantify a single protein in low concentrations
Example use cases
- Quantifying multiple protein biomarkers in one run with a multiplex assay.
- Monitoring proteins in plasma for disease progression and treatment effect.
- Detecting cytokines, chemokines, and growth factors, which are notoriously difficult to detect due to their low concentrations.
Innovations in immunoassay technologies offer higher sensitivity, accuracy, and efficiency
Digital ELISA: Taking sensitivity and flexibility to new levels
Digital ELISA allows researchers to dig deeper with higher sensitivity and less sample. Compared with traditional ELISA, Digital ELISA takes advantage of much, much smaller wells, those on the order of 15 femtoliters in volume. In this environment, a single molecule can produce a signal strong enough to be detected if the background noise is minimized. Additionally, if each well contains at most one molecule, and you can count up the number of wells that are giving a signal, then you have an extremely accurate measurement of the total number of target molecules in your sample.
Another significant advantage of this approach is its flexibility in terms plex and sample type. It’s capable of either single plex or multiplex detection, and because it can detect proteins at ultra low concentrations, researchers have their choice of sample type, be it blood, urine, serum, or cerebrospinal fluid (CSF).
The increased sensitivity and minimal sample volume allow for the detection of proteins that were difficult or impossible to measure in older types of immunoassays. The ability to customize and automate digital ELISAs offers a considerable advantage over traditional ELISAs and western blots because it reduces time and cost while quickly delivering a wealth of information.
Example use cases:
- Detect biomarkers of neuropathology to predict CSF profiles to stratify patients and potentially reduce the need for lumbar puncture Read the publication.
- Ultrasensitive Quantification of Cytokine Proteins in Individual Cells Read the publication.
- Measure brain-specific proteins in the blood to discriminate between Traumatic Brain Injury patients and controls Read the publication.
Western Blotting Using Capillary Electrophoresis (WesCE): Increasing efficiency through automation
Western blotting combined with capillary electrophoresis (CE) eliminates many of the problems associated with traditional western blotting. In this method, samples are prepared in glass capillaries containing stacking and separation matrices. Separated proteins are immobilized by covalent attachment to capillary walls, followed by immuno-probing and chemiluminescent detection.
One significant advantage of this technique is eliminating the need to transfer proteins to a membrane, reducing variation due to minor kinetic differences between runs — and avoiding the fretful scenario where your precious protein samples didn’t transfer.
The advantages don’t stop there. WesCE really shines when automated, where you simply load your samples, push a button, and return a few hours later or the next day to get your results. It can dramatically speed up time to results compared to traditional Western blotting protocols and gives you the gift of time to put your efforts elsewhere.
Example use cases
- Deepening cancer and immuno-oncology research through population level and single-cell level view of the tumor microenvironment
- Quantifying cell signaling analysis
- Investigating histone modifications in single nuclei to understand the epigenetic regulation of gene expression
Immunohistochemistry (IHC) Using Artificial Intelligence (AI): Expediting workflows and standardizing image analysis
Immunohistochemistry (IHC) is a powerful staining technique that utilizes a similar antibody-antigen binding process as ELISA but is applied to fresh or frozen tissue. Traditionally, IHC sample preparation and result interpretation is done manually and is therefore time consuming and subject to large variation.
Fortunately, there are solutions that automate various stages of the IHC protocol, reducing variability and standardizing data collection and processing. Among the most exciting developments is the analysis of IHC images using artificial intelligence (AI). After an initial training period, AI-aided software is able to differentiate protein-specific chromogenic labeling as well as nuclear counterstaining (Read more).
Applying machine learning and AI to IHC staining analysis has the potential to not only speed up workflows, but also to provide more accurate, reliable, and reproducible data analysis than manual methods. And the best part? The recent development of digital staining allow for an unlimited number of stains to be applied to just one sample.
Example use cases:
- Identifying and outlining tumor regions in cancerous tissue.
- Determining tissue distribution of an antigen of interest.
Questions to Answer When Selecting an Immunoassay
Quickly deciding which types of immunoassays to use is tempting, but it’s important to take the time to consider your experimental parameters and detection options first. If you choose the incorrect assay or label, you may waste time, precious sample, or worse, your analyte of interest might not be detected.
To help you make the right choice, let’s start by thinking through some key questions. Knowing the answers to these questions will help you choose the types of immunoassays and detection mechanism right for your lab and your research question.
Research Requirements
Start by answering these questions about your samples and research objectives.
- Sample type – What is your sample matrix? (serum, plasma, cell lysate, culture media, etc.)
- Number of samples – How many samples do you need to assess?
- Analyte type(s) – What analyte(s) do you need to measure?
- Number of target analytes – How many analytes do you need to detect?
- Analyte concentration – What is the expected concentration range of your sample? (pg/ml, ng/ml, µg/ml)
- Sensitivity – Do you need quantitative, semi-quantitative, or qualitative data?
- Reproducibility – How acceptable is variance between runs?
Resource Availability
Next, assess what is available to you.
- Sample volume – How much of each sample do you have to work with?
- Workflow – How much hands-on time can you spend? Is automation necessary?
- Available in-house platforms – What tools do you already have?
- Cost – Will immunoassays be a foundational approach in your experiments moving forward? Is immunoassay technology something your lab should consider investing in?
Immunoassay Technology Validation
Revisit these questions once you have selected an assay.
- Dynamic range – What is the antigen concentration range that the assay can accurately measure? Do you have enough sample to accommodate this?
- Validation – Has the immunoassay been validated for your target analyte and sample type?
The Future of Immunoassays with Quanterix
Immunoassay technology is always moving towards higher sensitivity, reproducibility, and data output combined with lower sample volumes. By using a microwell magnetic bead-based digital ELISA, Quanterix’s single molecule array (Simoa®) system does just that.
Because Simoa’s immunoassay technology can count single protein molecules, it boasts unmatched sensitivity that can measure proteins that are far below the lower limit of quantification in conventional immunoassay platforms, allowing the exploration and measurement of critical biomarkers that were previously out of reach. The Simoa system is fully automated, so there is limited manipulation of reagents and samples, reducing kinetic influences and improving reproducibility.
In addition to the broad dynamic range and the ease of multiplexing, Simoa utilizes small sample volumes and starts delivering results in as little as 30 minutes. Eager to check out ready-to-use Simoa kits and learn how Quanterix technology can revolutionize your research output and integrity?